A Google Maps for the Human Body
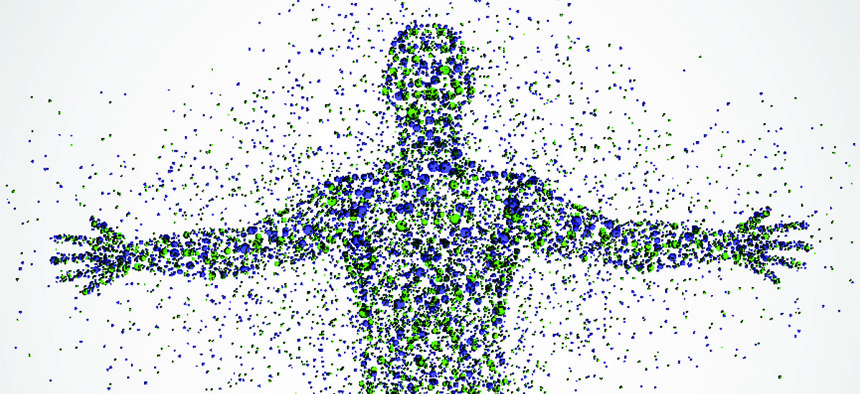
Lonely/Shutterstock.com
A group of scientists has taken the first important steps towards creating the Human Cell Atlas—a complete inventory of our staggeringly diverse cells.
Earlier this year, Steve McCarroll announced that his team had discovered the gene that most powerfully drives our risk of schizophrenia. Known as C4, it was previously viewed as an immune-system gene, but clearly, it also does something in the brain. To work out what, McCarroll first needed to know which cells in the brain activate C4.
Easier said than done: “There’s no place for looking that up,” he says. Instead, his team had to examine slices from over 700 postmortem brains, and stain them with a dozen different-colored antibodies that recognize C4. “The slices were variable in quality. The antibodies were variable in quality. It took us almost a year to get satisfying answers. It was a slog.”
McCarroll’s lament is a common one. Geneticists are constantly learning about genes that influence our risk of disease, but genes don’t perform in a vacuum. They perform in our cells. And since the 30 trillion cells in your body all share the same genes, you need to know which cells are actually using the gene in question. Where are those cells? What do they do normally, and what goes wrong in cases of disease? And in most cases, without the kind of slog that McCarroll endured, the answers are: we don’t know; no idea; and, ¯\_(ツ)_/¯.
Without such answers, a lot of the hype around biomedical advances becomes wishful thinking. With stem cells, scientists hope to re-grow damaged or lost tissues, but how do we regenerate something when we don’t entirely know what it’s composed of? With gene-editing techniques like CRISPR, we will supposedly hack diseases out of our DNA, but without deep knowledge of our cells, how do we know what to edit?
Our cells are the basic unit of our bodies—the stage upon which our genes enact their dramas. And simply put, “we really don’t know our cells.” says Aviv Regev from the Broad Institute. “And so we don’t know ourselves.”
Consider this fairly basic question: How many types of cells are there in the body? Around 200, according to several introductory pages from the National Institutes of Health. That figure corresponds to major groups, like neurons, heart cells, muscle cells, and more. But if you ask an immunologist, they’ll tell you there are at least 200 types of immune cells alone. Ask an immunologist who specializes in T-cells, and they’ll tell you there are at least 200 of those.
When I met Regev last year, she spent a good 15 minutes talking about all the variations within our cells. There are subtypes upon subtypes upon subtypes, she said. The retina alone contains at least 100 different classes of neurons. To complicate matters, some types of cells can transform into others. And each subtype can exist in many different states based on its environment, its neighbors, its position in a tissue, and the molecules it encounters.
"All of these things, the type and the subtype, the states, the locations, and the transitions … you’d want to know all of them," Regev told me. She let her words hang, and she smiled gently. Because she had a plan to do exactly that: to know everything.
Over the last few years, Regev has been slowly laying the foundations for compiling a Human Cell Atlas—a complete portrait of our cells in all their staggering diversity. It would list every subtype, how they change over time, where they are found, and which genes they switch on. Much like the first fully-sequenced human genome, it would be a resource so fundamental that biologists will use it many times a day without even thinking about it—a comprehensive, searchable Google Maps for the human body.
Creating such a resource is ambitious to say the least, and would have seemed impossible just five years ago. But thanks to technological advances, Regev and others think that the time is right. “The idea has been bubbling,” says Sarah Teichmann from the Wellcome Trust Sanger Institute. “I had talked with someone about this four years ago. At the time, it seemed a bit crazy. Even now, it seems crazy—but also more feasible. And people are willing to consider it.”
That’s partly due to Regev, who has been evangelizing for the atlas since 2014. She is widely respected, and not just for her boundless energy and fierce intellect. “She’s one of the smartest people I’ve ever met, but also intense, perceptive, compassionate and caring,” says Dana Pe’er from Columbia University.
Case in point: Last June, at a meeting, Regev noticed that Pe’er was suffering from back pain, surreptitiously emailed her to ask if she was okay, and texted a colleague to bring in a more comfortable chair—all while leading a debate between big-name scientists and summarizing their discussion in real time. “The people sitting right next to me didn’t notice a thing,” says Pe’er. “Aviv did, from the other side of the room with a bad field of view.”
Yesterday, in London, Regev and Teichmann convened another meeting of like-minded scientists who were interested in their vision of a cell atlas. The event was effectively a soft launch—not of the atlas itself, but of a budding community that will actually start making it happen.
They already have a funding commitment from the Chan-Zuckeberg Initiative—the recently formed company that is aiming to “cure, prevent or manage all disease by the end of this century.” “The Human Cell Atlas would be a transformative technology that advances all of science,” CZI President Cori Bargmann told the assembled researchers. “We’re pretty much on board with saying that this should happen, and we want to help make it happen with the people in this room.”
As is often the way in science, Regev’s dream for the Human Cell Atlas began with a simple surprise.
When scientists study cells, they typically smoosh a large population of them together. They get a clear portrait of an average cell, but they whitewash all the diversity within the group. To unveil that diversity, Regev and others had been developing hardware and software for studying single cells. She first turned those tools onto a group of immune cells called dendritic cells back in 2012, isolating just 18 of these cells and cataloguing all the genes that they were activating.
“We were immediately astounded,” she says. It turned out that 3 of those 18 cellswere switching on very different sets of genes than the others. What was supposedly a pure sample of identical cells turned out to be a pool of two distinct types. “It was as if we thought that everyone in a city wore green shirts, and it turned out that some wore blue and some wore yellow.”
She repeated the study with over 1,700 dendritic cells, and found even more variation. Dendritic cells are watchmen: they grab molecules that could be signs of infectious threats, and present those molecules to the rest of the immune system for inspection. But in response to the same molecules, seemingly identical cells would react by switching on different genes. Some did so much earlier than others, and then instigated the responses of their more apathetic neighbors.
These experiments showed Regev how much she and other scientists were missing. “All of these years, when we did these studies, we would grow millions of cells in a dish and pretend they were one,” she says. “We’d take these average measurements and say: A cell! We knew that wasn’t correct, but you work with what you have.”
But technology has changed, in both power and affordability. It cost Regev thousands of dollars to analyze just those 18 dendritic cells in 2012. By 2013, with some robotics, she had taken the price down to ten bucks a cell.
McCarroll, who also works at the Broad Institute, pushed the cost down even further. He and his team developed a technique called DropSeq, which sends cells down extremely narrow canals, single file, and incarcerates them in droplets of oil. Each oily cage is a millionth of a milliliter in volume, and contains precisely one prisoner. Once in solitary confinement, the cells can be split open and their DNA can be sequenced. So can their RNA—a related molecule that reveals which genes are being switched on.
With DropSeq, Regev and McCarroll can simultaneously study hundreds of thousands of single cells, at just 6 cents each. They can start doing the work it would take to actually build a Human Cell Atlas in a cost-effective way. “Aviv has been pushing the cutting edge to do things that almost seem impossible, and she's made unbelievable ground in a very short period of time,” adds Pe’er. “I didn't think it could be done that fast.”
The Human Cell Atlas isn't just an academic cataloguing exercise. It might be the key to making the promises of biomedicine real.
When geneticists identify genes behind diseases, they want to understand where those genes are active. When immunologists engineer cells to attack cancers based on the molecules on their surface, they'd better know whether healthy cells somewhere in the body naturally harbor those same molecules. And when tissue engineers dream of growing new organs in a lab, they need to know if what they're producing actually matches their natural counterparts.
The Human Cell Atlas isn’t going to reveal everything about our bodies, just as the Human Genome Project didn’t tell us everything about our genes. But it should provide a foundational resource—something that spurs countless future experiments and makes those experiments easier. “Think of what having the genome has done for biology: We can find genes that cause disease, and sequence tumors, and personalize medicine,” says Pe’er. “I think the Human Cell Atlas would have the same impact. And we need it to make sense of our genome.”
It might also help scientists to make more use of finished research. Researchers have already sequenced the DNA of countless tissue samples, taken from people with cancer and all kinds of other conditions. Regev can look at those sequences and, with a few computational tricks, reverse-engineer which types of cells were present.
She has already tried this with melanoma skin cancers. “We can say this tumor pretty much only had malignant cells, or this one had a lot of immune cells,” she says. “There’s so much hidden there that we don’t yet know.” That might help cancer biologists to better classify tumors into distinct types, and develop more effective tailor treatments—all without collecting anything new. “These samples come from people who had diseases,” she adds. “We owe them and the taxpayers to learn the maximum amount we can.”
Skeptics might argue that tech aside, the Human Cell Atlas is still implausible. The Human Genome Project had an obvious endpoint—produce a near-complete genome. But cataloguing our cells seems like fighting a Hydra—find one subtype and two more rise to take its place. Regev thinks otherwise. “Is every cell going to be its own special snowflake? I would say not,” she says. “They group into stable categories.”
The task is huge, but neither infinite nor insurmountable. And at the very least, the atlas initiative will tell us how big it is. Put it this way: our knowledge of our cells is currently like a blurry map of the world, with the outlines of continents and oceans but little else. The Human Cell Atlas will offer better resolution—it will map the position of all the mountains and rivers, even if every single rock and stream isn't known.
Indeed, Regev has already been running pilot projects to show that the atlas is both feasible and valuable. She can identify all the major known cell types in the retina of a mouse, and has even found a few new ones—an astonishing feat given how well-studied that tissue is. She's now looking at tumors, immune cells, the gut, and two parts of the brain.
She’s not alone. Many other groups of scientists are running similar projects using their own techniques for analyzing single cells. Smaller-scale atlases are already underway. The BRAIN Initiative—the Obama administration’s bold plan to understand the brain—included a census of cell types as its first goal. “Individual projects are taking bite-size chunks out of this problem,” says Richard Conroy from the National Institute of Biomedical Imaging and Bioengineering. “The question is how to pool all this together into a complete picture.”
Yesterday’s meeting was the first step. The gathered researchers included several pioneers of single-cell genomics—Pe’er, Stephen Quake of Stanford University,Sten Linnarson from Stockholm’s Karolinska Institute, and many others—who are now firmly on board with the Atlas initiative. Together, they started hashing out its goals and details. Which organs should they look at first? How do they get samples from places like the pancreas, which are very hard to reach? Which techniques should they use? They talked technology and tactics. They shared opinions about obstacles and opportunities. And most of all, they got to know each other—which is exactly what Regev and Teichmann wanted.
The Atlas endeavor will “generate an international community of scientists who will know each other and continue to work with each other beyond the project itself,” said Mike Stratton from the Wellcome Trust Sanger Institute, who opened the meeting. “Many will be young and their lifelong scientific perspectives will be shaped by this initiative. What we’re starting off today will be creating a new field of human biology. That’s an immensely inspiring aim.”
“At first people will say it’s impossible,” adds Teichmann. “Later, they’ll say it was obvious.”