'Digital Human' Teaches How to Walk and Protect Your Knees
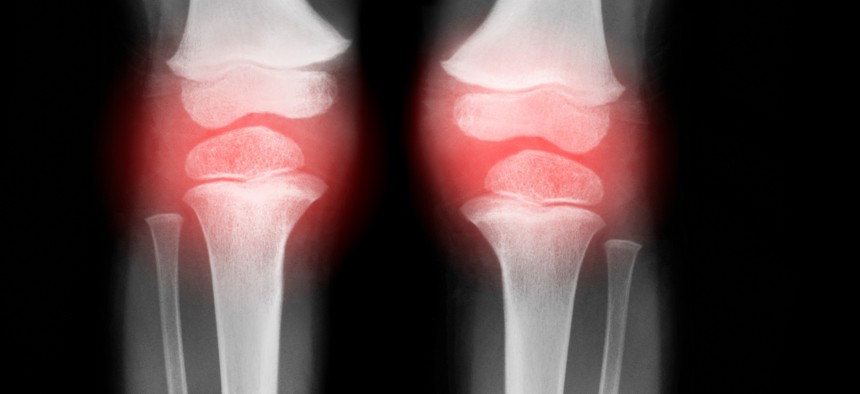
Peter Dazeley/Getty
A digital person might have some teaching advantages over actual people.
Researchers have created a “digital human” that shows how to reduce force on the knee by teaching people to use different muscles as they walk.
Using results from the digital human, a detailed computer simulation, participants in a small study were able to reduce the load on their knees by an average of 12%, a benefit equivalent to a person losing about 20% of their total body weight. The lighter load may alleviate pain from osteoarthritis or prevent joint injuries.
“We now have sufficiently realistic mathematical and computational models of human movement that we can change how the brain excites muscles in a simulation, and see how that affects joint loads,” says Scott Delp, professor of bioengineering at Stanford University, director of the Wu Tsai Human Performance Alliance, and senior author of the study in Scientific Reports.
“For instance, if your knee hurts, how can we change the forces on your knee so you feel better?” he says.
‘MORE MUSCLES THAN WE NEED TO WALK’
Knee pain is rampant: Almost a quarter of Americans age 45 and older suffer from osteoarthritis of the knee, and knee pain accounts for nearly 4 million visits to primary care physicians each year.
“We’ve known for a while that the majority of force that compresses the knee is actually caused by muscle forces, from muscles that cross the knee and generate force when they contract,” says lead author Scott Uhlrich, a research engineer at Stanford.
“IF YOU HOOKED YOUR ACHILLES TENDON TO THE BACK OF A SMALL CAR, EXCITING THOSE MUSCLES COULD ACTUALLY LIFT THE CAR.”
As a person walks, the force pushing their bones together in the knee is equivalent to two to four times their body weight; during running or playing sports, force on the knee is even higher. This repetitive compression can wear away at the cartilage, causing osteoarthritis.
Traditional treatments for osteoarthritis include weight loss, knee bracing, and joint replacement, but none of these treatments target the muscle forces, Uhlrich says.
Unlike a robot, which is built with a single motor to power each joint, humans have multiple muscles crossing their knees, ankles, and hips.
“We have way more muscles than we need to walk,” Uhlrich says, “which gives our brain a lot of options for which muscles to use.”
For example, two different calf muscles—the gastrocnemius and the soleus—can do the same action of pushing the foot down, but only the gastrocnemius crosses the knee and creates a compression force.
“People don’t realize how strong the forces from these muscles are,” Delp says. “For instance, if you hooked your Achilles tendon [which attaches the gastrocnemius and the soleus to the heel] to the back of a small car, exciting those muscles could actually lift the car.”
All that force can be damaging to the knee. But with the digital human, the researchers were able to find muscle coordination strategies that generated less force on the knee joint. They discovered that by increasing activation of the soleus muscle and decreasing activation of the gastrocnemius muscle, they could drastically reduce force on the knee without changing a person’s gait.
MUSCLE RELIEF
What the researchers didn’t know was whether real people could use these muscle coordination strategies during a complex task like walking. “You might not think of walking as a complicated activity,” Delp says, “but there’s a lot going on under the hood.”
Each time the brain tells a muscle to move, it sends a small electrical signal that can be measured in the muscle through electromyography (EMG), similar to the way an electrocardiogram measures electrical activity in the heart.
Previous studies showed that giving participants a visual representation of their muscle activity—EMG biofeedback—could help them relax those muscles, but only while sitting still and doing a simple task, such as moving a finger up and down. No one had tested it for walking.
Acting as his own first test subject, Uhlrich attached EMG electrodes to his leg muscles while walking on a treadmill so he could visualize the muscles being used. “At first I tried it with just the raw EMG data,” he says, “but the patterns were way too complicated. I realized we needed to simplify the feedback.”
After much experimentation, the researchers settled on a single bar graph that would teach people to reduce activation of their gastrocnemius muscle, while increasing the use of their soleus muscle.
“As soon as I was able to simplify the signal and do it successfully myself, I got Dr. Delp onto the treadmill,” Uhlrich says. “When he did it pretty quickly as well, we got excited and brought in more people for the study.”
With each step, participants tried to shrink the bar on a graph showing the ratio of their gastrocnemius-to-soleus muscle activation. After just five minutes of this biofeedback, all 10 participants in the study were able to significantly reduce the use of their gastrocnemius compared with their soleus while walking.
When researchers removed the biofeedback and tested whether participants could retain the new muscle coordination strategy after six minutes of walking without biofeedback, 8 out of 10 participants were able to do so, resulting in decreased load on the knee in six of these cases.
“We were amazed,” Delp says. “We really didn’t know if we could train people to do this, which is what made the discovery so exciting.”
TRAINING AND WEARABLE BIOFEEDBACK
Because the initial study included only healthy volunteers, the next step will be to test the muscle coordination strategies in patients with osteoarthritis to determine whether the reduced force on the knee translates into a reduction in pain and other symptoms. Another question: How long after a biofeedback session can the participants maintain the new pattern of muscle activation?
Making the changes permanent would likely require more than one or two sessions, Delp says. “To fully incorporate the new coordination pattern into your daily life, you may need a wearable device that gives you feedback for a month or so,” he says.
The researchers have recently applied for a patent and are planning to coordinate with a bioengineering company to build a wearable feedback system.
In conjunction with personalized digital human simulations, wearable biofeedback could revolutionize not just treatment for osteoarthritis, but all kinds of joint pain, including overuse injuries in athletes. “There are a lot of cases where if we were to activate our muscles differently, we could reduce stress on important structures,” Uhlrich says. For example, baseball pitchers are prone to ligament tears in their elbows, which can require career-ending surgery.
The researchers imagine a future in which baseball pitchers could come into a lab, have a personalized simulation of their elbow made, and use that “digital athlete” to identify muscle coordination strategies to protect their ligaments. Then they could go home with a wearable biofeedback device that would retrain their muscles to prevent injuries.
“With the digital human, you can try anything,” Delp says. “And when you combine it with what we discovered in this paper—that you can teach people new strategies to coordinate their muscles—it opens so many doors.”
The National Institutes of Health, a fellowship from the National Science Foundation, and the Sang Samuel Wang Stanford Graduate Fellowship from the Stanford Office of the Vice Provost for Graduate Education funded the work.
Researchers have created a “digital human” that shows how to reduce force on the knee by teaching people to use different muscles as they walk.
Using results from the digital human, a detailed computer simulation, participants in a small study were able to reduce the load on their knees by an average of 12%, a benefit equivalent to a person losing about 20% of their total body weight. The lighter load may alleviate pain from osteoarthritis or prevent joint injuries.
“We now have sufficiently realistic mathematical and computational models of human movement that we can change how the brain excites muscles in a simulation, and see how that affects joint loads,” says Scott Delp, professor of bioengineering at Stanford University, director of the Wu Tsai Human Performance Alliance, and senior author of the study in Scientific Reports.
“For instance, if your knee hurts, how can we change the forces on your knee so you feel better?” he says.
‘MORE MUSCLES THAN WE NEED TO WALK’
Knee pain is rampant: Almost a quarter of Americans age 45 and older suffer from osteoarthritis of the knee, and knee pain accounts for nearly 4 million visits to primary care physicians each year.
“We’ve known for a while that the majority of force that compresses the knee is actually caused by muscle forces, from muscles that cross the knee and generate force when they contract,” says lead author Scott Uhlrich, a research engineer at Stanford.
As a person walks, the force pushing their bones together in the knee is equivalent to two to four times their body weight; during running or playing sports, force on the knee is even higher. This repetitive compression can wear away at the cartilage, causing osteoarthritis.
Traditional treatments for osteoarthritis include weight loss, knee bracing, and joint replacement, but none of these treatments target the muscle forces, Uhlrich says.
Unlike a robot, which is built with a single motor to power each joint, humans have multiple muscles crossing their knees, ankles, and hips.
“We have way more muscles than we need to walk,” Uhlrich says, “which gives our brain a lot of options for which muscles to use.”
For example, two different calf muscles—the gastrocnemius and the soleus—can do the same action of pushing the foot down, but only the gastrocnemius crosses the knee and creates a compression force.
“People don’t realize how strong the forces from these muscles are,” Delp says. “For instance, if you hooked your Achilles tendon [which attaches the gastrocnemius and the soleus to the heel] to the back of a small car, exciting those muscles could actually lift the car.”
All that force can be damaging to the knee. But with the digital human, the researchers were able to find muscle coordination strategies that generated less force on the knee joint. They discovered that by increasing activation of the soleus muscle and decreasing activation of the gastrocnemius muscle, they could drastically reduce force on the knee without changing a person’s gait.
MUSCLE RELIEF
What the researchers didn’t know was whether real people could use these muscle coordination strategies during a complex task like walking. “You might not think of walking as a complicated activity,” Delp says, “but there’s a lot going on under the hood.”
Each time the brain tells a muscle to move, it sends a small electrical signal that can be measured in the muscle through electromyography (EMG), similar to the way an electrocardiogram measures electrical activity in the heart.
Previous studies showed that giving participants a visual representation of their muscle activity—EMG biofeedback—could help them relax those muscles, but only while sitting still and doing a simple task, such as moving a finger up and down. No one had tested it for walking.
Acting as his own first test subject, Uhlrich attached EMG electrodes to his leg muscles while walking on a treadmill so he could visualize the muscles being used. “At first I tried it with just the raw EMG data,” he says, “but the patterns were way too complicated. I realized we needed to simplify the feedback.”
After much experimentation, the researchers settled on a single bar graph that would teach people to reduce activation of their gastrocnemius muscle, while increasing the use of their soleus muscle.
“As soon as I was able to simplify the signal and do it successfully myself, I got Dr. Delp onto the treadmill,” Uhlrich says. “When he did it pretty quickly as well, we got excited and brought in more people for the study.”
With each step, participants tried to shrink the bar on a graph showing the ratio of their gastrocnemius-to-soleus muscle activation. After just five minutes of this biofeedback, all 10 participants in the study were able to significantly reduce the use of their gastrocnemius compared with their soleus while walking.
When researchers removed the biofeedback and tested whether participants could retain the new muscle coordination strategy after six minutes of walking without biofeedback, 8 out of 10 participants were able to do so, resulting in decreased load on the knee in six of these cases.
“We were amazed,” Delp says. “We really didn’t know if we could train people to do this, which is what made the discovery so exciting.”
TRAINING AND WEARABLE BIOFEEDBACK
Because the initial study included only healthy volunteers, the next step will be to test the muscle coordination strategies in patients with osteoarthritis to determine whether the reduced force on the knee translates into a reduction in pain and other symptoms. Another question: How long after a biofeedback session can the participants maintain the new pattern of muscle activation?
Making the changes permanent would likely require more than one or two sessions, Delp says. “To fully incorporate the new coordination pattern into your daily life, you may need a wearable device that gives you feedback for a month or so,” he says.
The researchers have recently applied for a patent and are planning to coordinate with a bioengineering company to build a wearable feedback system.
In conjunction with personalized digital human simulations, wearable biofeedback could revolutionize not just treatment for osteoarthritis, but all kinds of joint pain, including overuse injuries in athletes. “There are a lot of cases where if we were to activate our muscles differently, we could reduce stress on important structures,” Uhlrich says. For example, baseball pitchers are prone to ligament tears in their elbows, which can require career-ending surgery.
The researchers imagine a future in which baseball pitchers could come into a lab, have a personalized simulation of their elbow made, and use that “digital athlete” to identify muscle coordination strategies to protect their ligaments. Then they could go home with a wearable biofeedback device that would retrain their muscles to prevent injuries.
“With the digital human, you can try anything,” Delp says. “And when you combine it with what we discovered in this paper—that you can teach people new strategies to coordinate their muscles—it opens so many doors.”
The National Institutes of Health, a fellowship from the National Science Foundation, and the Sang Samuel Wang Stanford Graduate Fellowship from the Stanford Office of the Vice Provost for Graduate Education funded the work.if